1. What is vegetal chitosan?
Chitosan is a natural biopolymer that is primarily derived from chitin, which is the second most abundant natural polysaccharide after cellulose. Chitin is commonly found in the exoskeletons of crustaceans like crabs, shrimps, and lobsters, as well as in the cell walls of certain fungi like mushroom, aspergillus niger.
Structure and Properties:
- Chemical Structure: Chitosan is obtained by deacetylating chitin. This process involves removing acetyl groups from chitin, resulting in a polymer that has free amine groups.
- Solubility: Unlike chitin, chitosan is soluble in acidic to neutral solutions, making it more versatile for various applications.
- Biocompatibility and Biodegradability: Chitosan is known for its excellent biocompatibility and biodegradability. It’s non-toxic and can be broken down by natural biological processes.
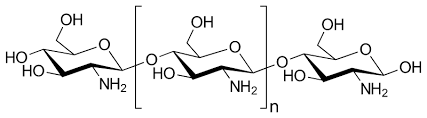
Chitosan, sometimes known as deacetylated chitin, is a natural polycationic linear polysaccharide derived from partial deacetylation of chitin. Chitin is the structural element in the exoskeleton of insects, crustaceans (mainly shrimps and crabs shell), and cell walls of fungi (oyster mushroom, agaricus bisprous and aspergillus niger), and also is the second most abundant natural polysaccharide after cellulose.

Chitosan, a natural polysaccharide prepared of fungal origin, is initially extracted and purified from reliable and abundant food or biotechnological fungal sources such as Agaricus bisporus or Aspergillus niger.
Chitosan is composed of glucosamine sugar units (deacetylated units) and N-acetyl-D-glucosamine units (acetylated units) interconnected by ß→(1.4) type linkages.
Uses and Applications:
- Medical and Pharmaceutical Fields: Due to its biocompatibility and non-toxic nature, chitosan is used in wound healing, drug delivery systems, and as a biomaterial in tissue engineering.
- Water Treatment: Its ability to bind with heavy metals and other contaminants makes it useful in water purification and treatment processes.
- Agriculture: As a natural biostimulant and elicitor, chitosan is used to enhance plant growth and provide resistance against pathogens.
- Food Industry: It’s used as a food additive for its antimicrobial properties, and as an edible film or coating to enhance the shelf life of perishable food products.
- Cosmetics and Personal Care: In this realm, it finds use as a thickener, moisturizer, and film-forming agent, particularly in hair and skin care products.

Chitosan has been widely used in various fields, including wine, pharmaceuticals, dietary supplement, medicine, agriculture, and food industries, due to its biocompatibility, biodegradability, and non-toxicity. In recent years, researchers have investigated the use of vegetal chitosan, which is derived from fungal or plant sources, as a sustainable alternative for use in wine applications.
Variants:
- Traditional Chitosan: Traditionally sourced from marine crustaceans.
- Vegetal Chitosan: Derived from fungal sources, offering an alternative for those seeking non-animal derived products.
In summary, chitosan’s versatility, biodegradability, and non-toxic nature make it a valuable material across various industries, from healthcare to cosmetics. Its ability to be derived from non-animal sources also makes it an appealing option for vegetarian and vegan-friendly products.
2. What are the advantages of vegetal chitosan?
Vegetal chitosan, also known as fungal chitosan or mycelium chitosan, is a type of chitosan derived from the cell walls of fungi (mushroom and aspergillu niger). It has several advantages over traditional chitosan derived from shellfish, including:

- 1. Vegan and vegetarian-friendly: Vegetal chitosan is an excellent alternative for individuals who avoid animal-based products, such as those who follow a vegan or vegetarian lifestyle.
- 2. Allergen-free: Traditional chitosan is derived from shellfish, which can cause allergic reactions in some people. Vegetal chitosan does not contain any shellfish-derived ingredients, making it an allergen-free option.
- 3. Purer: Vegetal chitosan is often considered to be purer than traditional chitosan because it is derived from a single source, whereas traditional chitosan can be contaminated with other shellfish-related substances.
- 4. Better solubility: Vegetal chitosan is more soluble than traditional chitosan, which makes it easier to incorporate into various applications such as cosmetics, pharmaceuticals, wine and food.
- 5. Improved bioavailability: Some studies have suggested that vegetal chitosan has a higher bioavailability compared to traditional chitosan, which means that it can be absorbed and utilized more effectively by the body.
Overall, vegetal chitosan offers several advantages over traditional chitosan, making it an attractive alternative for individuals and industries looking for a vegan, allergen-free, and more effective chitosan source.
3. What are the benefits & functions of vegetal chitosan in 3D Printing application?
Vegetal chitosan sourced from mushrooms or Aspergillus niger offers several benefits and functions when used in 3D printing applications. Here are some of the key advantages:
- Biocompatibility and Biodegradability: Vegetal chitosan is highly biocompatible and biodegradable, making it an excellent choice for medical applications such as scaffolds for tissue engineering, biodegradable implants, or drug delivery systems.
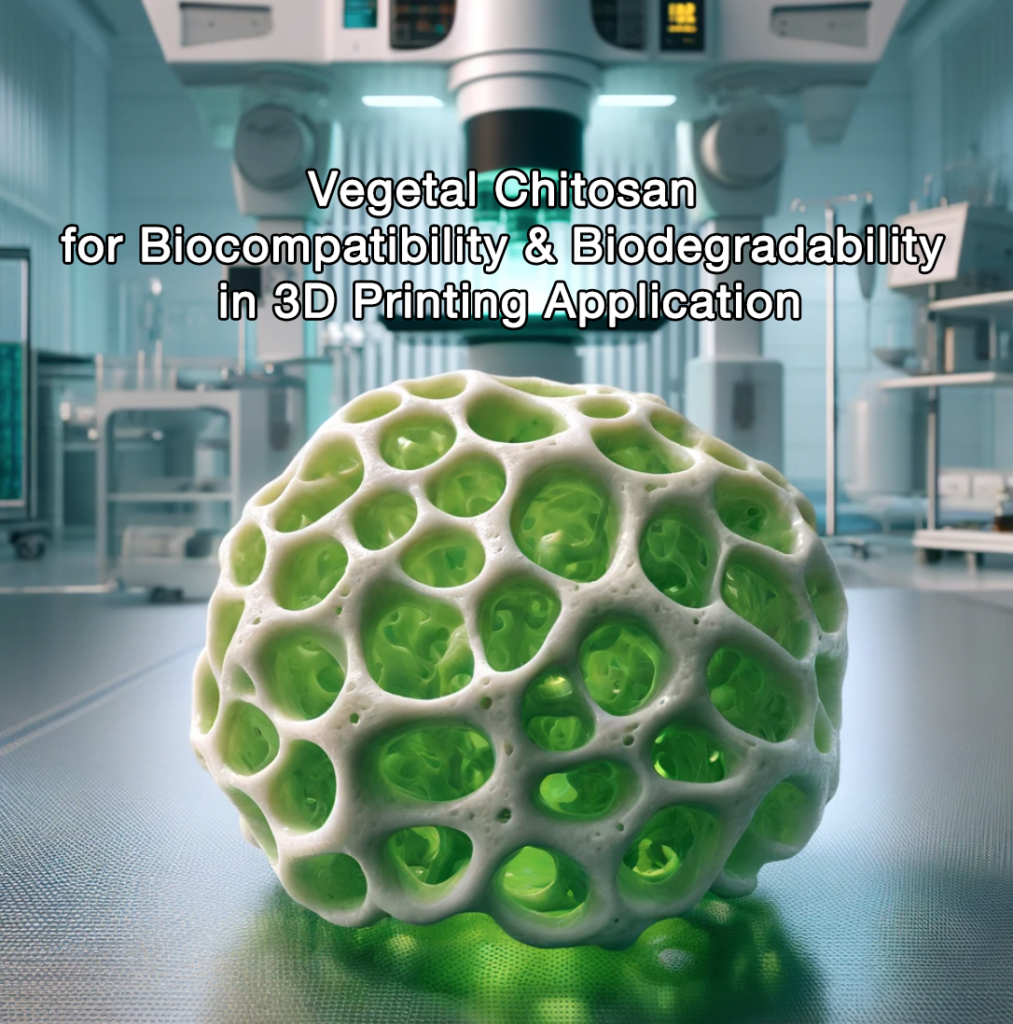
- Mechanical Strength: The addition of vegetal chitosan to biopolymer blends used in 3D printing can enhance the mechanical properties of the printed objects. This includes improved tensile strength and elasticity, which are crucial for creating durable and functional items.
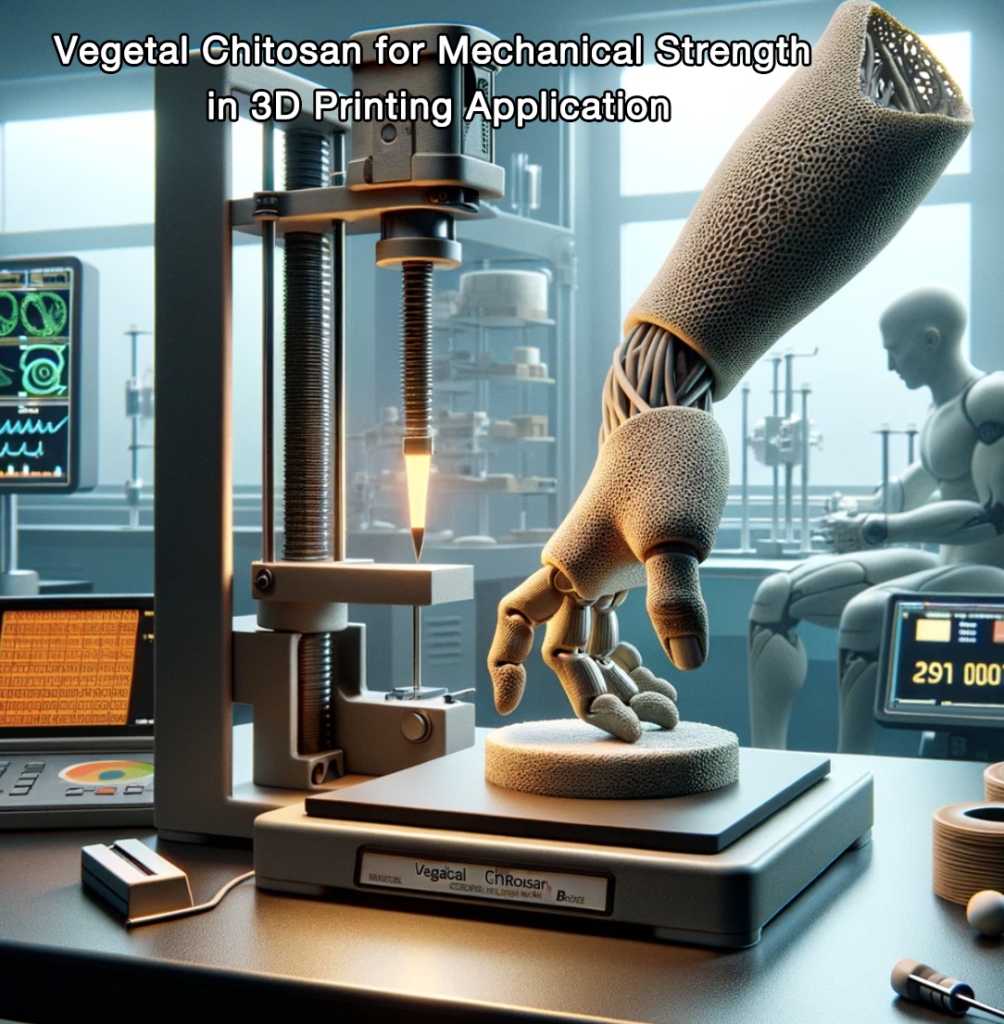
- Antimicrobial Properties: Chitosan is well known for its antimicrobial properties. Incorporating vegetal chitosan into 3D printing filaments can impart antibacterial and antifungal characteristics to the printed objects, making them suitable for medical devices and other applications where sterility is crucial.
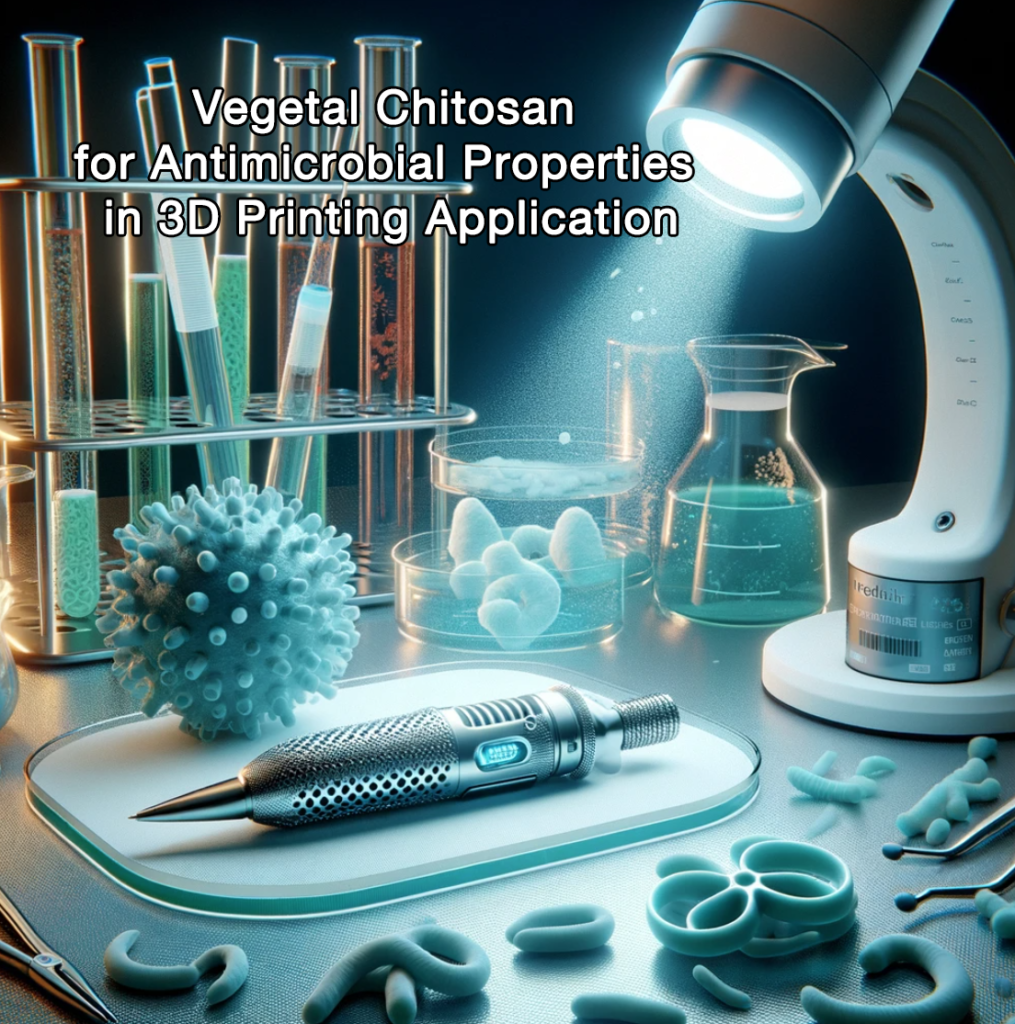
- Support for Cell Growth: In biomedical applications, vegetal chitosan can support cell adhesion and growth, making it ideal for creating 3D printed scaffolds that promote tissue regeneration.
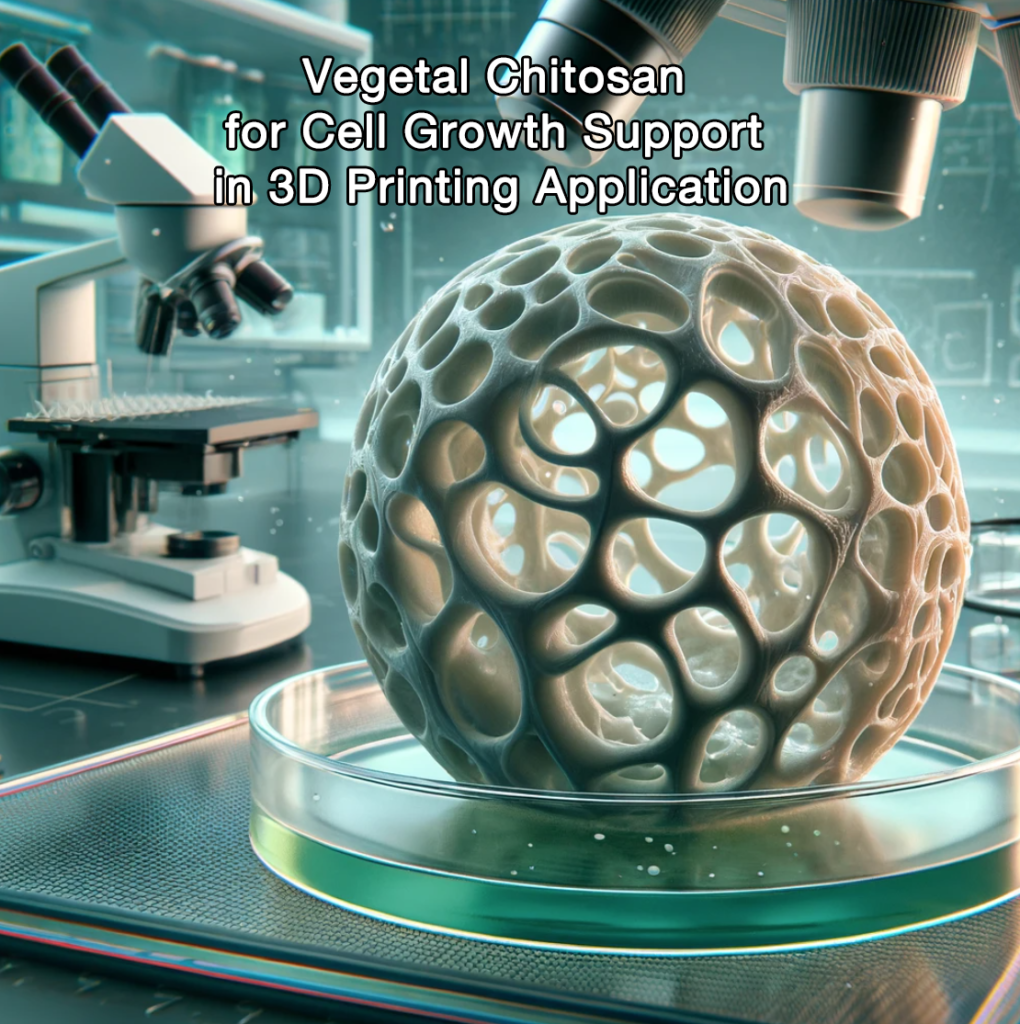
- Environmental Impact: Being derived from mushrooms or fungi like Aspergillus niger, vegetal chitosan is a more sustainable option compared to traditional plastic materials. It contributes to reducing the environmental footprint of 3D printing.
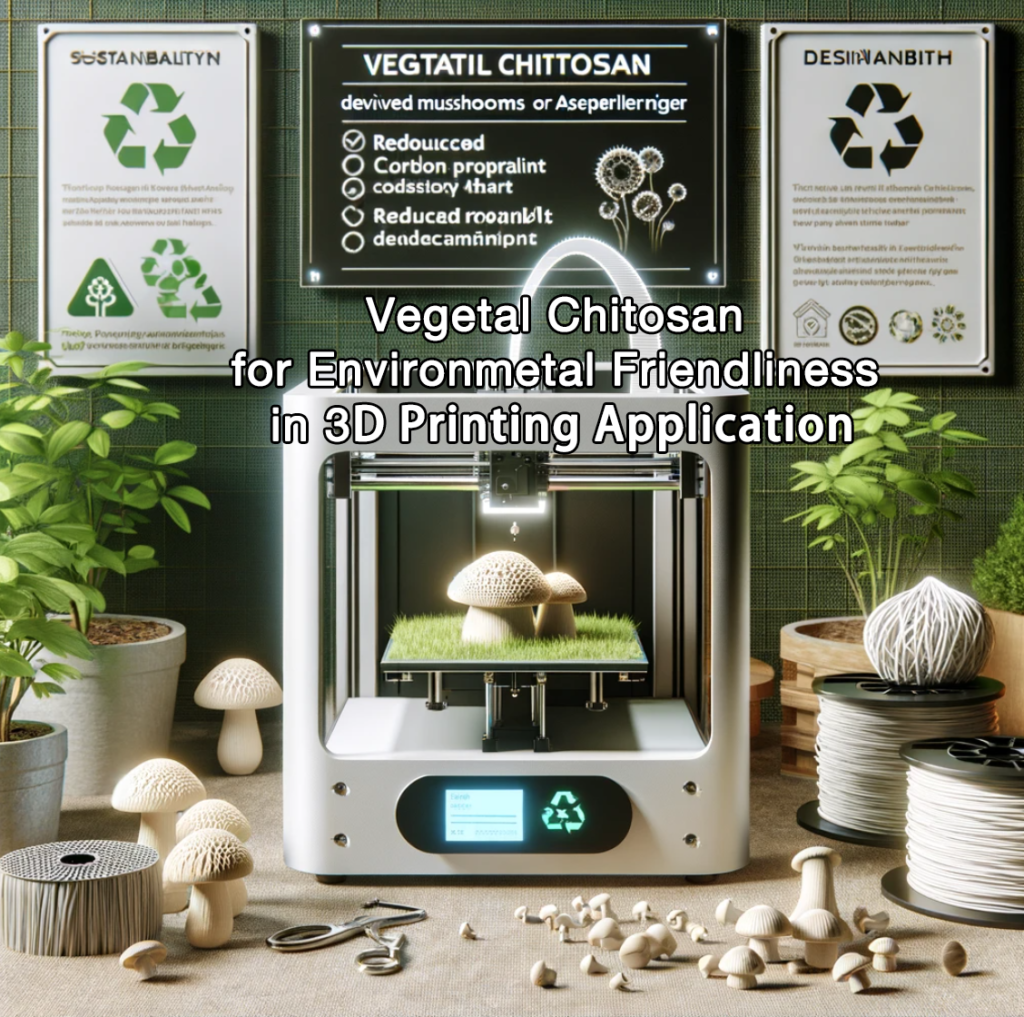
- Chemical Functionalization: Chitosan’s chemical structure allows for easy functionalization, meaning that it can be chemically modified to achieve specific properties desired in the final printed product. This adaptability makes it useful in creating specialized materials for targeted applications.
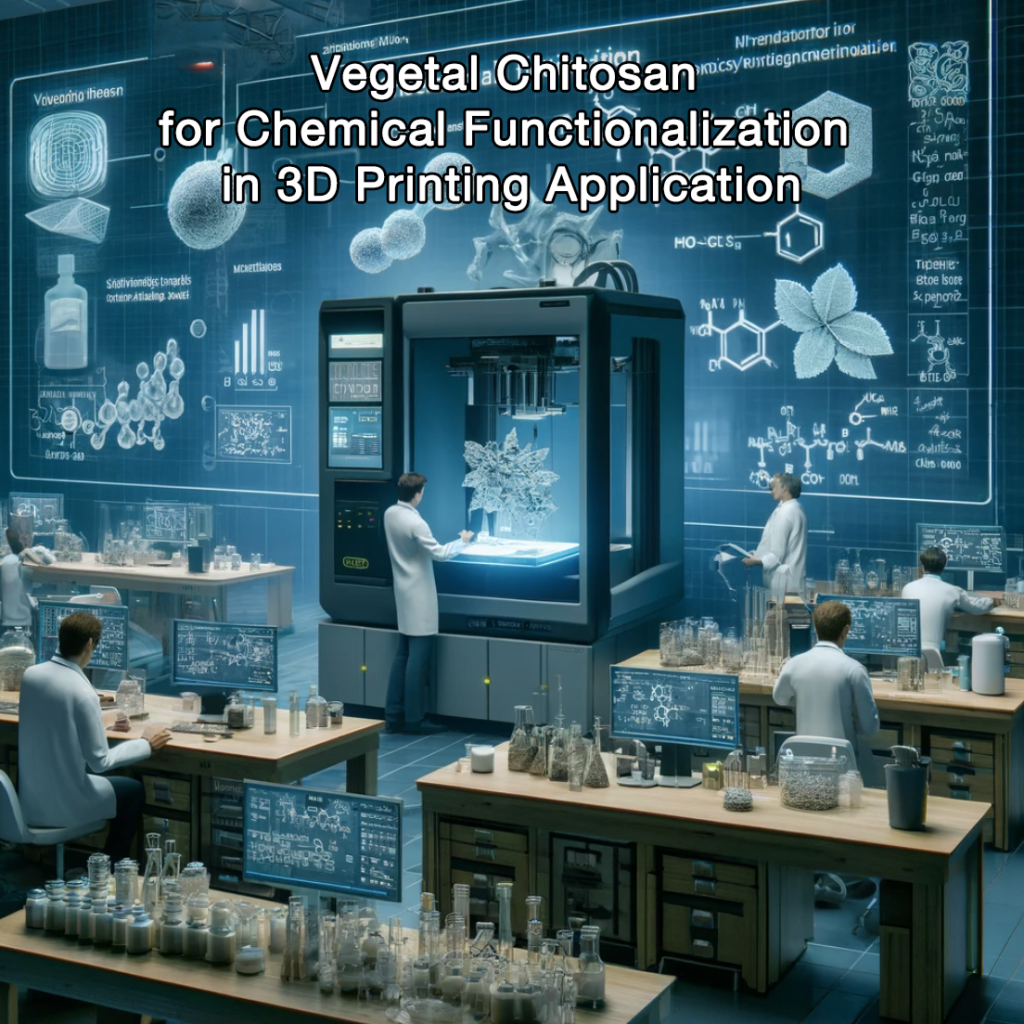
These attributes make vegetal chitosan a versatile and valuable material in the field of 3D printing, particularly for applications that require biocompatibility, environmental sustainability, and functional performance.
4. Which specific areas & products of 3D printing application vegetal chitosan can be used?
Vegetal chitosan, derived from sources like mushrooms or Aspergillus niger, offers unique properties that make it particularly useful in various specific areas and products of 3D printing.
And the optimal addition ratio of chitosan in 3D printing applications depends on the specific properties and functionality required for each product.
Here are some of the key applications and products where vegetal chitosan can be effectively utilized, along with a general guide on how chitosan might be incorporated into various 3D printing applications and products:
- Biomedical Scaffolds: Vegetal chitosan is ideal for fabricating 3D printed scaffolds used in tissue engineering. These scaffolds support the growth and regeneration of tissues such as bone, cartilage, and skin.
- Bone Regeneration Scaffolds: 3D printed scaffolds that facilitate the growth and integration of new bone tissue.
- Cartilage Repair Structures: Custom structures to support cartilage regeneration in joints.
- Dermal Scaffolds: Used for skin regeneration, particularly in treating burns and large wounds.
- Chitosan Addition Ratio: Chitosan is typically used in concentrations of 1% to 3% by weight in composite materials that may also include bioceramics like hydroxyapatite or other polymers to enhance structural and mechanical properties. The exact ratio can vary based on the required scaffold porosity and mechanical strength.
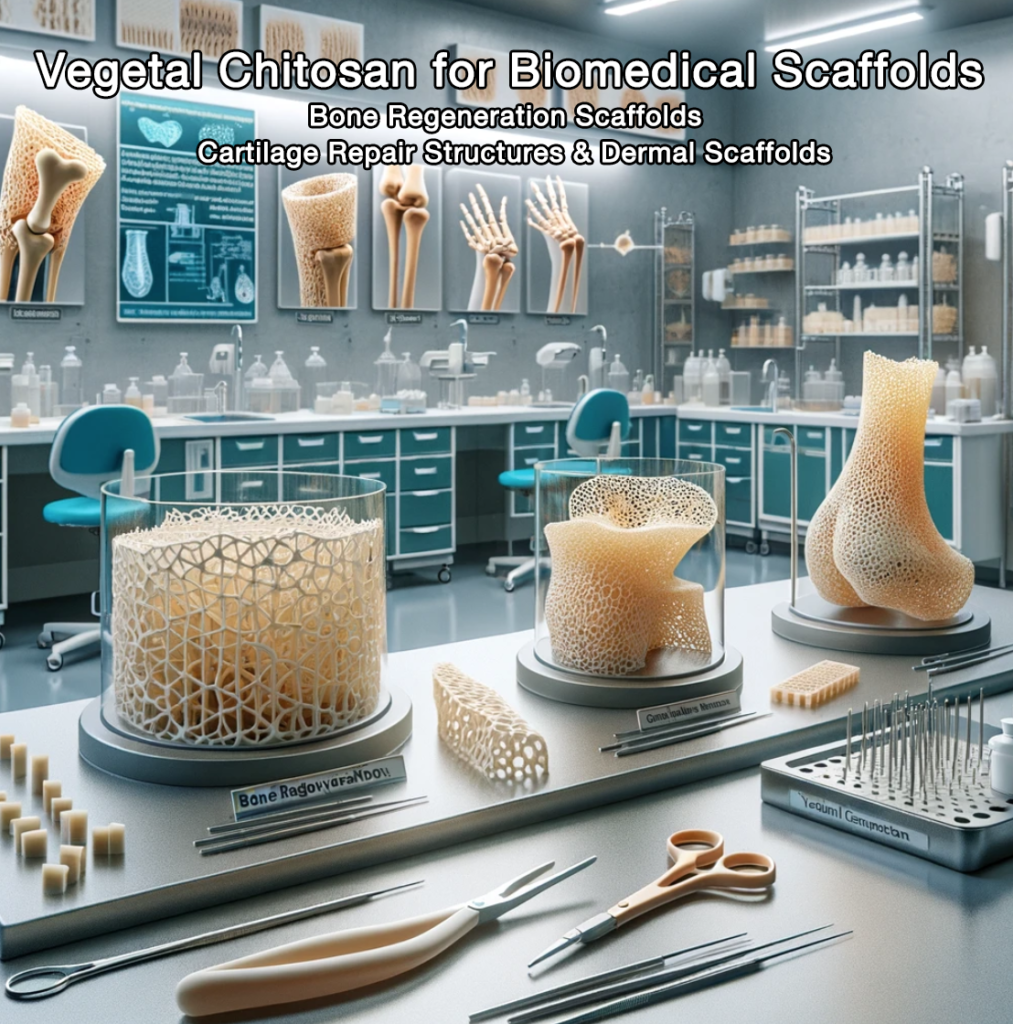
- Drug Delivery Devices: Due to its biocompatibility and ability to be chemically modified, vegetal chitosan can be used to create 3D printed structures for targeted and controlled drug delivery systems.
- Controlled Release Tablets: Tablets designed to release drugs at controlled rates.
- Localized Cancer Treatment Implants: Implants that slowly release chemotherapy agents directly at the tumor site.
- Chitosan Addition Ratio: For drug delivery applications, chitosan might constitute 1% to 5% of the formulation, often blended with other polymers or active ingredients. The concentration will depend on the desired rate of drug release and the method of printing.
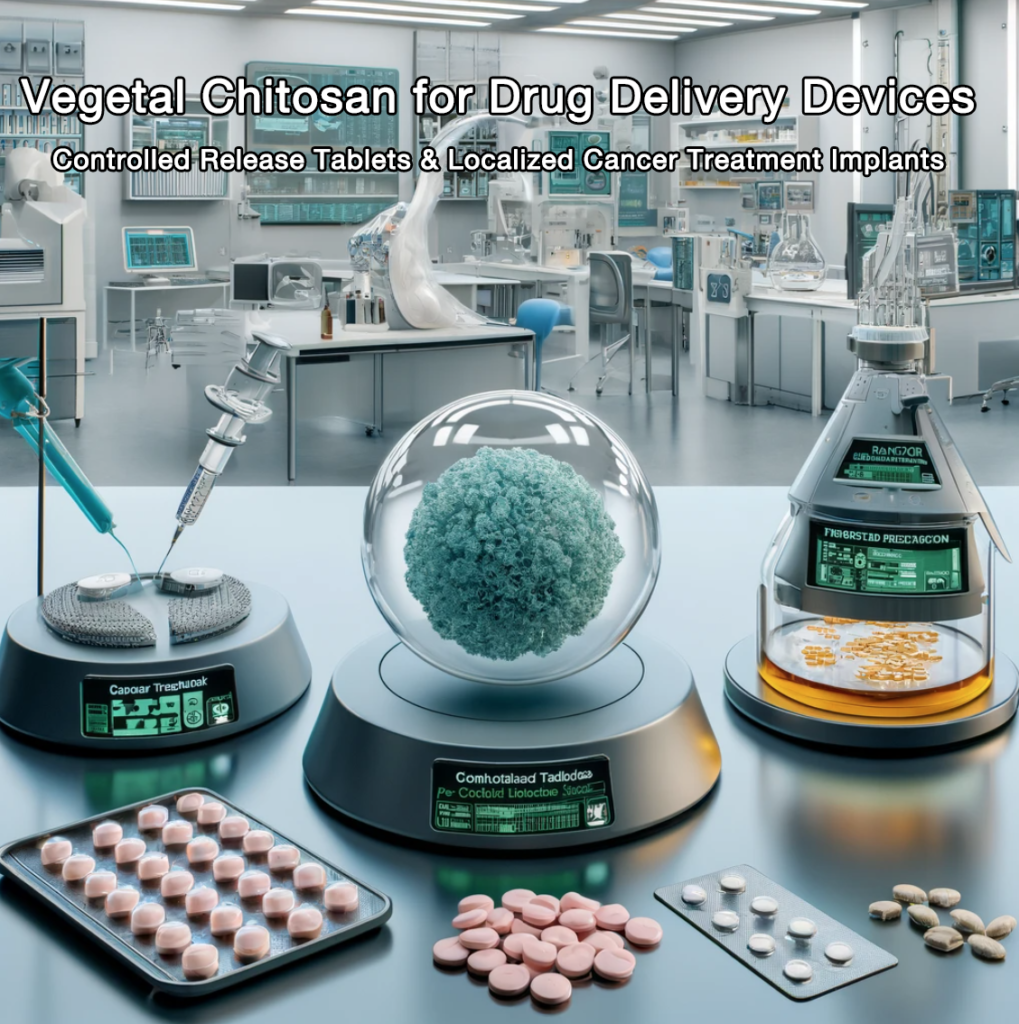
- Dental Implants and Structures: In dentistry, 3D printed chitosan can be used to create models and implants that are customized to the patient’s anatomy, improving the outcomes of various dental treatments.
- Custom Dental Implants: Personalized implants that match the patient’s oral geometry.
- Periodontal Disease Treatments: Structures that release therapeutic agents to treat gum disease.
- Chitosan Addition Ratio: In dental applications, chitosan is used in a range from 2% to 10%, depending on the need for structural integrity and the specific printing technology used. Higher concentrations may be used to improve printability and mechanical properties.
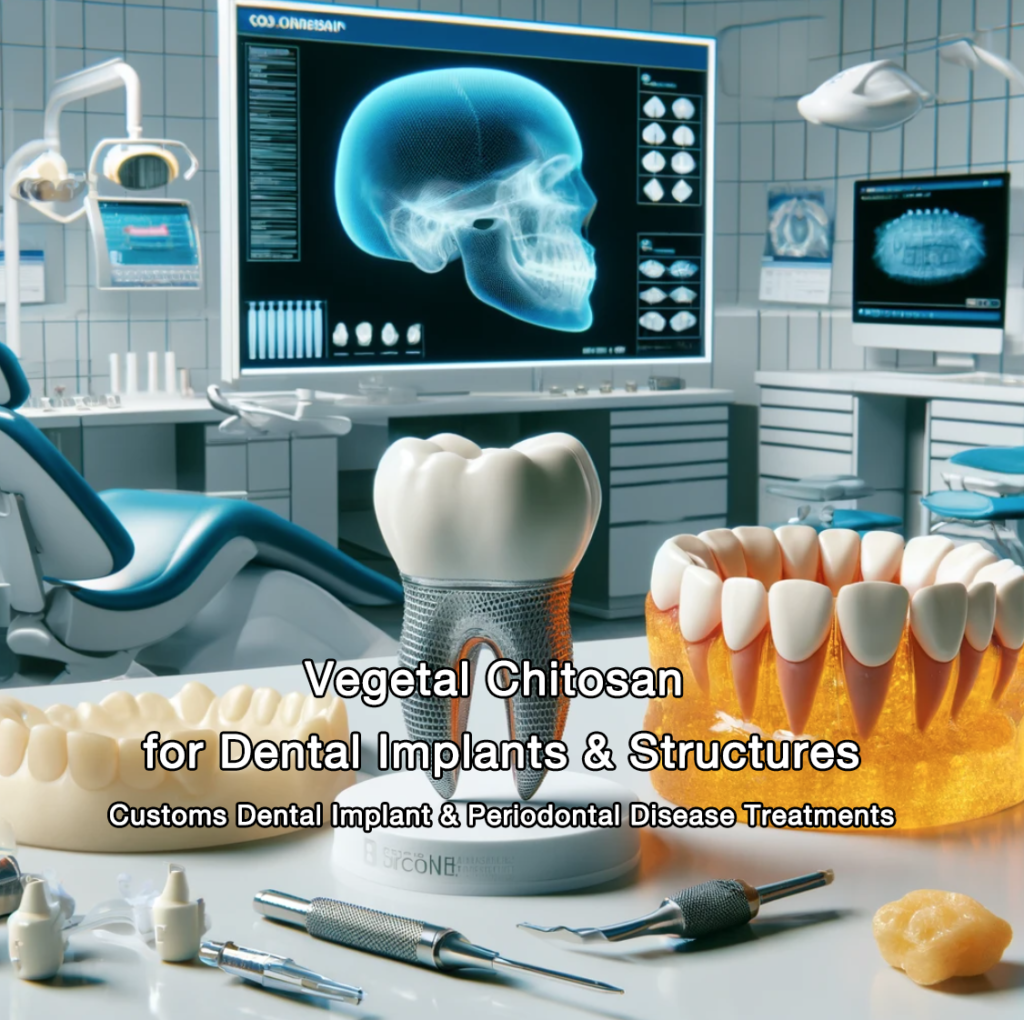
- Customized Prosthetics: Vegetal chitosan can be used in the production of biodegradable and biocompatible prosthetics, customized to the specific needs of patients.
- Biodegradable Prosthetic Limbs: Custom-fitted limbs that are lightweight and tailored to the user’s specific measurements.
- Cosmetic Prosthetics: Prosthetics designed for aesthetic purposes, such as facial reconstruction.
- Chitosan Addition Ratio: For prosthetics, chitosan might be blended at 5% to 20% with other biocompatible materials to ensure sufficient mechanical strength and durability, alongside enhancing biodegradability.
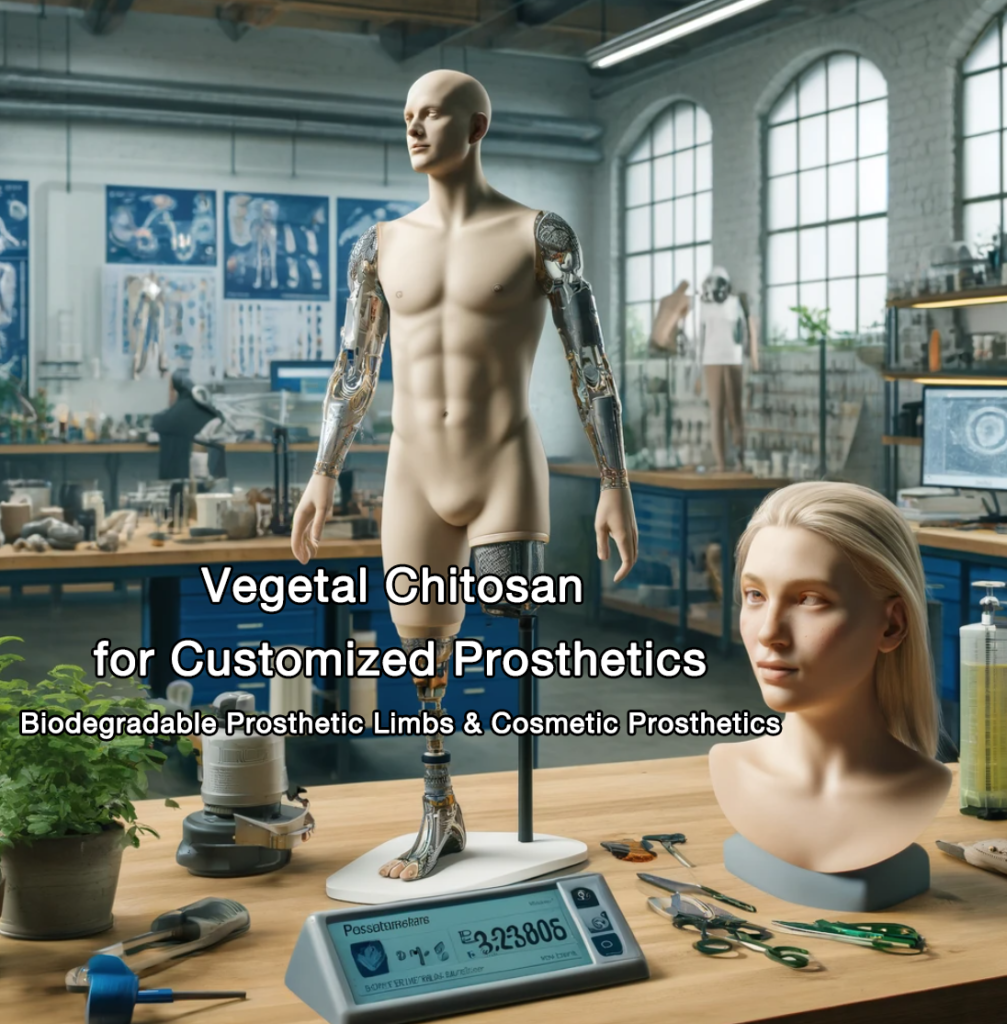
- Surgical Planning and Training Models: 3D printed models using chitosan can mimic human tissue characteristics, making them excellent tools for surgical preparation and educational purposes.
- Anatomical Models for Surgery Rehearsal: Highly accurate replicas of patient-specific anatomy used for pre-surgical planning and training.
- Educational Models for Medical Training: Models used in medical schools for teaching anatomy and surgical techniques.
- Chitosan Addition Ratio: Chitosan usage can vary widely, from 1% to 10%, often mixed with other materials to adjust the model’s rigidity and fidelity to human tissue characteristics.

- Wound Healing Products: Chitosan’s natural antimicrobial and healing properties make it suitable for 3D printing wound dressings and other products that facilitate faster healing of wounds.
- Antimicrobial Wound Dressings: Dressings that prevent infection and promote healing.
- Skin Healing Patches: Patches that deliver growth factors directly to the wound site, accelerating tissue regeneration.
- Chitosan Addition Ratio: Chitosan is often used at 1% to 3% for hydrogel formulations or higher concentrations for films and foams, depending on the required thickness and moisture retention properties.
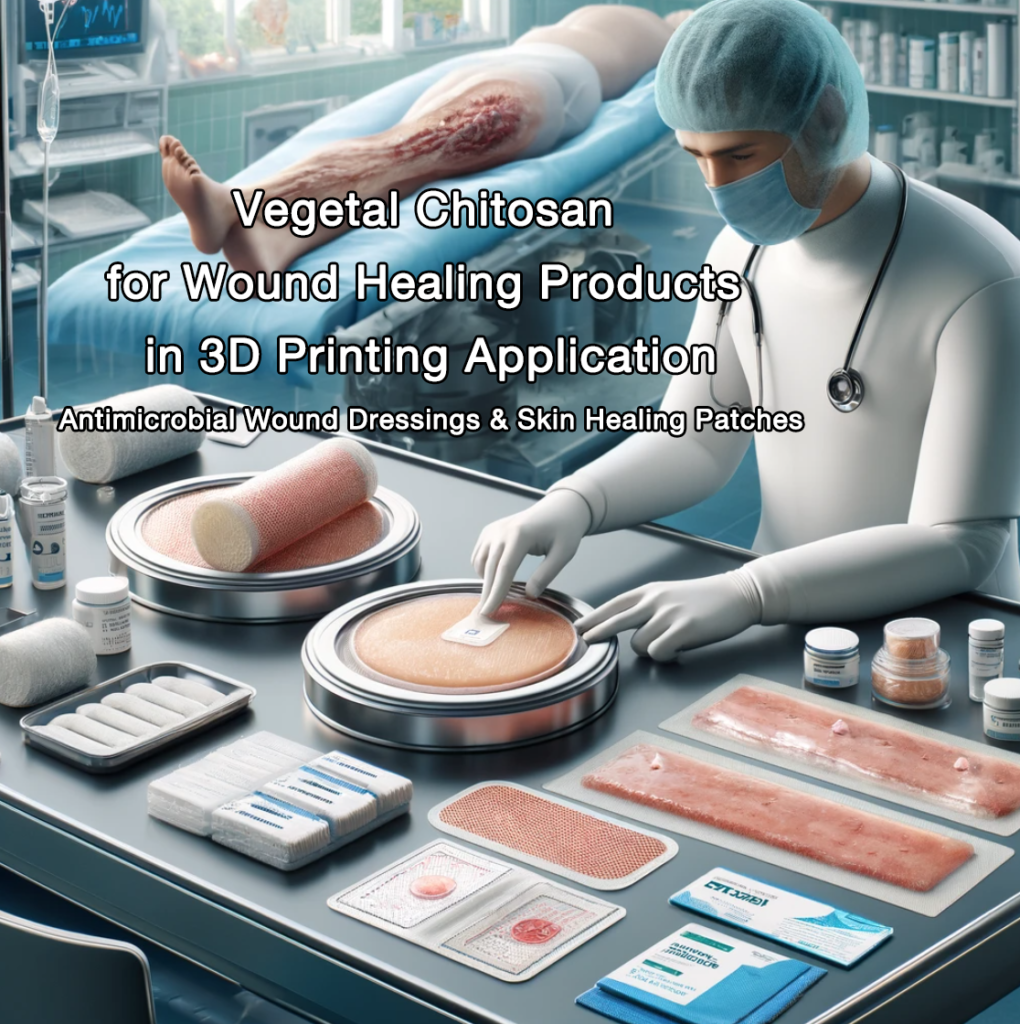
- Implant Coatings: Chitosan coatings on 3D printed implants can improve their integration with biological tissues, enhancing the healing process and reducing the risk of infection.
- Anti-Infection Implant Coatings: Coatings that release antimicrobial agents to reduce the risk of post-surgical infections.
- Biocompatible Coatings for Enhanced Integration: Coatings designed to improve the integration of implants with body tissues, reducing rejection rates.
- Chitosan Addition Ratio: Typically, a thin coating of chitosan, ranging from 0.5% to 2% solutions, is used to enhance the antimicrobial properties and biocompatibility of implants.
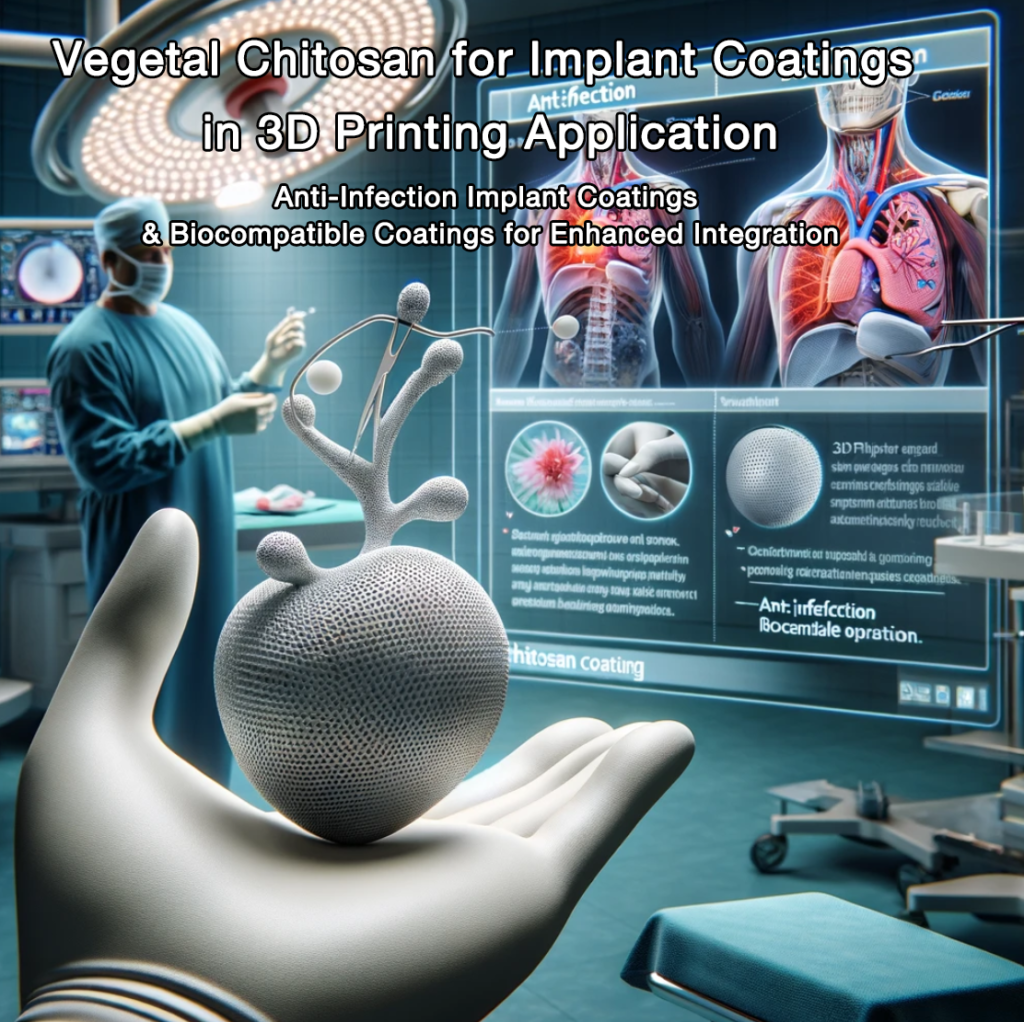
These applications exploit the biodegradable, biocompatible, and functionalizable nature of vegetal chitosan, making it a valuable material in the 3D printing industry, especially in fields requiring stringent standards for safety and compatibility.
And these products leverage the unique properties of vegetal chitosan, such as biodegradability, biocompatibility, and the capacity to support cellular activities, making them particularly suitable for advanced medical applications where traditional materials may fall short.
Moreover, these ratios are indicative and can vary based on the specific application, the desired properties of the final product, the type of 3D printing technology (e.g., extrusion-based printing, resin printing, powder bed fusion), and the processing conditions. Optimization through experimentation and prototyping is common to achieve the desired outcomes in terms of functionality and performance.
5. Which type of chitosan is more suitable for each application area or products in 3D printing?
For 3D printing applications, the choice of chitosan type significantly influences the processing ability and the quality of the final product. Each type of chitosan mentioned offers different properties that make them suitable for specific 3D printing applications:
- Acid-Soluble Chitosan (viscosity 20-100 cps):
- Drug Delivery Devices: Suitable for creating intricate devices with fine details, as lower viscosity aids in precision printing.
- Wound Healing Products: Ideal for thin, flexible layers needed in advanced wound dressings, allowing for easy application and comfort.
- Acid-Soluble Chitosan (viscosity 100-500 cps):
- Biomedical Scaffolds: Provides a good balance between flowability and structural integrity, essential for creating scaffolds that support cell growth and tissue formation.
- Dental Implants and Structures: Offers sufficient viscosity for the detailed printing required for dental applications while maintaining ease of processing.
- Acid-Soluble Chitosan (viscosity 500-1000 cps):
- Customized Prosthetics: Higher viscosity helps in forming more robust and durable prosthetics, which need to withstand mechanical stress.
- Surgical Planning and Training Models: Benefits from higher viscosity to ensure stability and detail retention in complex anatomical models.
- Chitosan Hydrochloride:
- Implant Coatings: Its enhanced solubility makes it ideal for creating coatings on implants that can be easily applied and uniformly cover complex geometries.
- Drug Delivery Systems: Useful for creating drug delivery mechanisms that require precise control over the release rates, aided by its solubility characteristics.
- Chitosan Oligosaccharide:
- Skin Care Products: Low molecular weight and high solubility are advantageous for 3D printed cosmetic applications, allowing for better skin absorption.
- Nutraceutical Delivery: Suitable for printing delivery systems for bioactive compounds that benefit from rapid dissolution and absorption.
- Carboxymethyl Chitosan:
- Wound Dressings: Its hydrophilic nature makes it excellent for creating moisture-maintaining dressings, crucial for healing environments.
- Biomedical Scaffolds: Enhances cell attachment and proliferation due to its modified surface, making it ideal for tissue engineering applications.
Each type of chitosan offers unique advantages depending on the requirements of the application, such as solubility, viscosity, biocompatibility, and mechanical properties, making the selection process critical to the success of the 3D printed products.
6. What are the common form of vegetal chitosan used in 3D printing?
In 3D printing, vegetal chitosan is utilized primarily in forms that are amendable to the printing process and the desired properties of the final product.
Each type of chitosan offers unique properties that make it particularly suitable for different 3D printing applications.
Here are the common forms in which vegetal chitosan is used, and a detailed breakdown of recommended application areas for each form of chitosan:
- Chitosan Powder: This is the most basic form of chitosan, which can be dissolved in suitable solvents (like acetic acid solutions) to prepare the printing ink or filament. It allows for control over viscosity and concentration, which are critical for achieving the desired properties in the printed structure.
- Surgical Models and Prototyping: The powder form can be tailored in terms of viscosity for detailed and precise model creation, suitable for surgical rehearsals and educational purposes.
- Customized Drug Delivery Systems: Allows for the inclusion of various pharmaceutical agents, adjusting the powder’s formulation for specific release profiles.
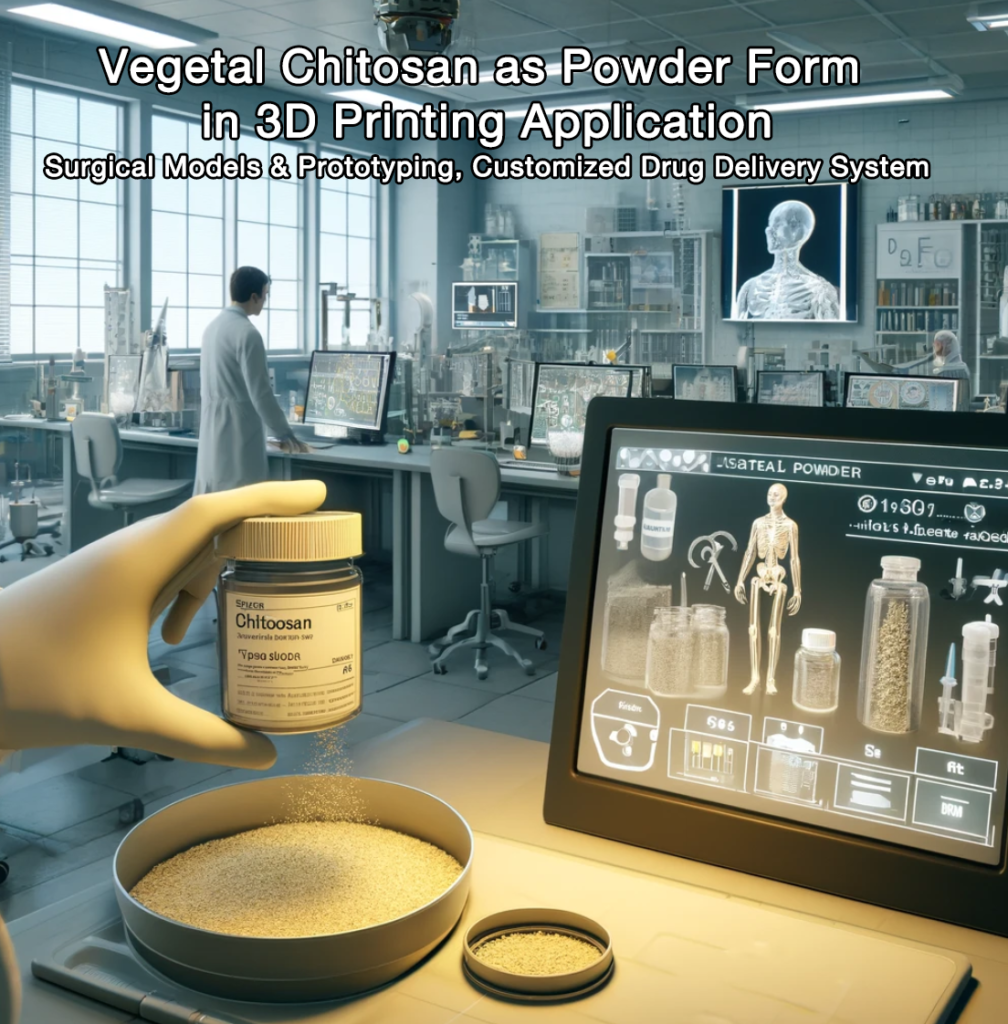
- Chitosan Solutions: Pre-prepared chitosan solutions are commonly used in 3D printing for their ease of use. These solutions can be directly loaded into the 3D printer if compatible with the printer’s specifications. The concentration and viscosity of these solutions are adjusted based on the printing requirements.
- Wound Healing Applications: Solutions can be used to print hydrogel-based dressings that conform to wound sites and release medicinal agents to promote healing.
- Tissue Engineering Scaffolds: These solutions can be printed into scaffolds that support cell growth, particularly in applications requiring high precision and uniformity in pore size and structure.
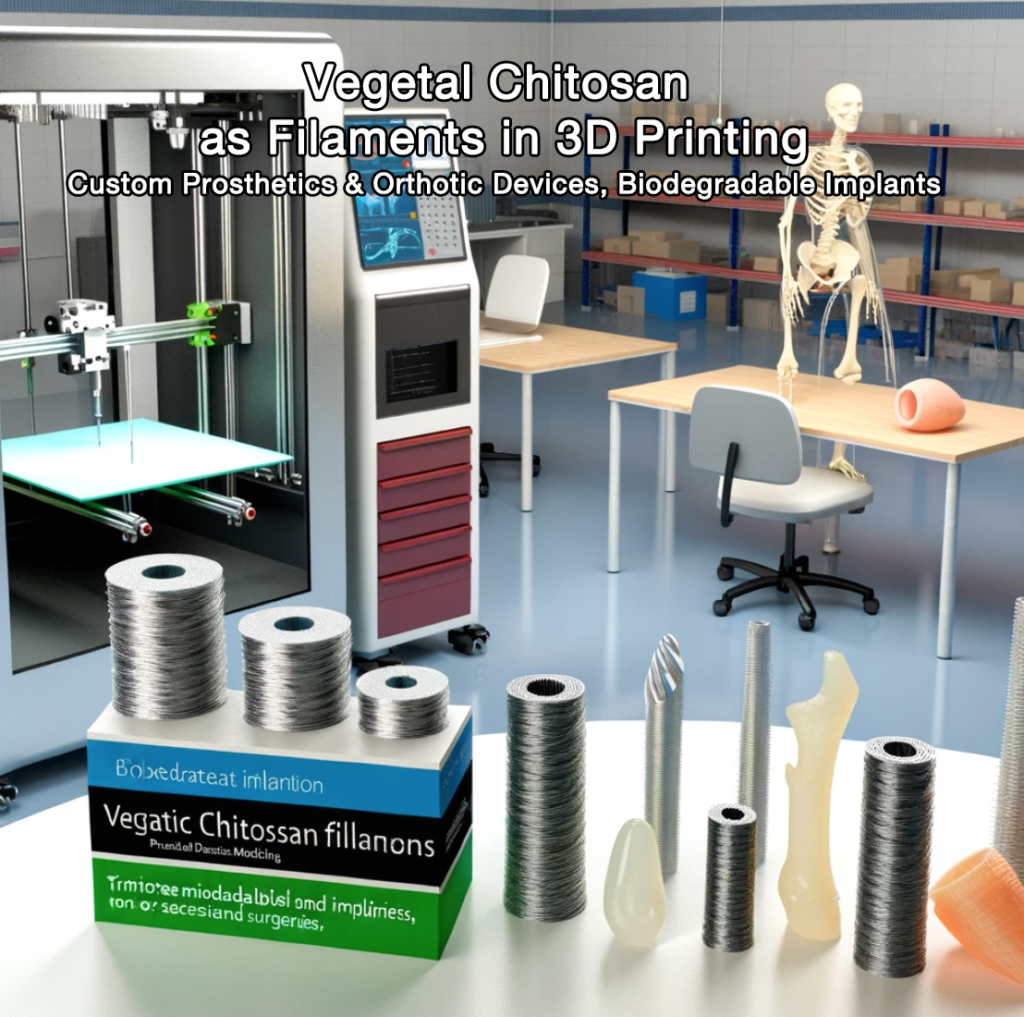
- Chitosan Filaments: For certain types of 3D printers, especially those that use fused deposition modeling (FDM), chitosan can be processed into filaments. These are typically composite materials where chitosan is blended with other biopolymers to improve its mechanical properties and printability.
- Custom Prosthetics and Orthotic Devices: Filaments are ideal for creating durable and body-compatible prosthetic and orthotic devices that are custom-fitted to the patient.
- Biodegradable Implants: Suitable for printing implants that slowly degrade and are absorbed by the body, reducing the need for secondary surgeries to remove implants.
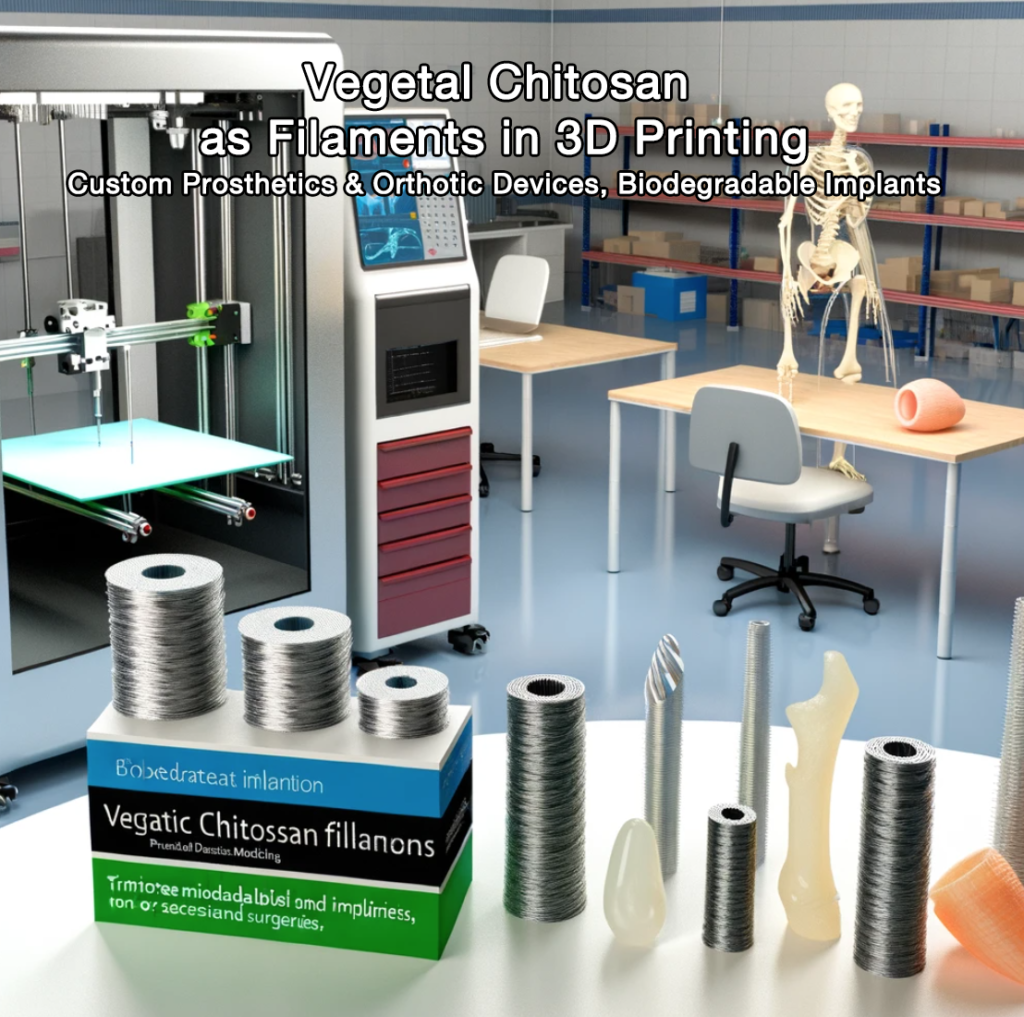
- Chitosan Hydrogels: Hydrogels are particularly useful in applications requiring high water content and soft structures, such as tissue engineering scaffolds. Chitosan hydrogels can be cross-linked to provide structural stability and maintain their shape post-printing.
- Soft Tissue Engineering: Perfect for creating scaffolds that mimic the natural soft tissue environment, supporting the cultivation of delicate tissues like liver, lung, or cardiac tissues.
- Cell Culture Media: Hydrogels can be used as 3D matrices for cell culture, providing a supportive environment that mimics the natural extracellular matrix.
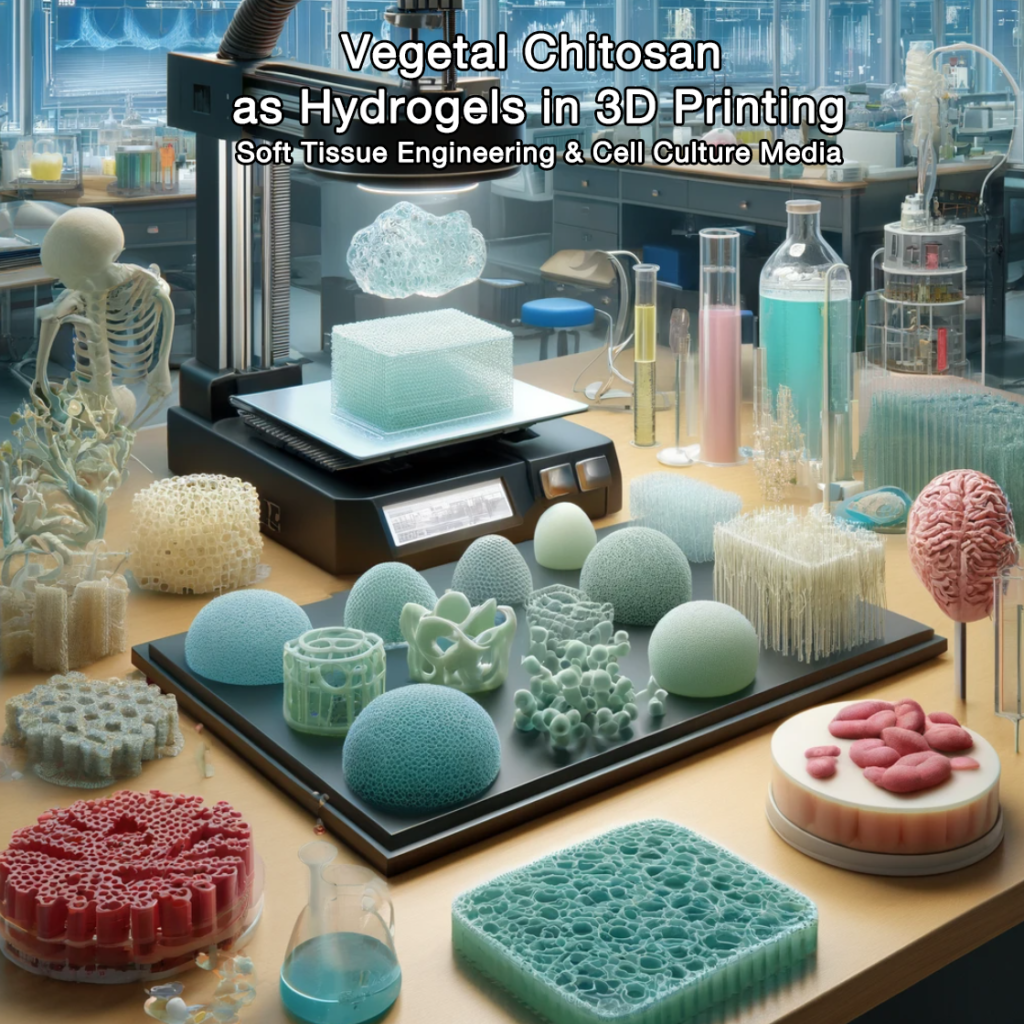
- Chitosan-Based Composite Materials: Chitosan is often combined with other materials, such as bioceramics (e.g., hydroxyapatite), other biopolymers, or nanoparticles, to enhance the properties of the final product. These composites are tailored for specific applications, like bone tissue engineering, where enhanced biocompatibility and mechanical strength are needed.
- Bone Tissue Engineering: Composites can incorporate bioceramics like hydroxyapatite, enhancing the mechanical strength and osteoconductivity needed for bone regeneration.
- Dental Applications: Ideal for printing dental implants and scaffolds that require enhanced mechanical properties and biocompatibility with oral tissues.
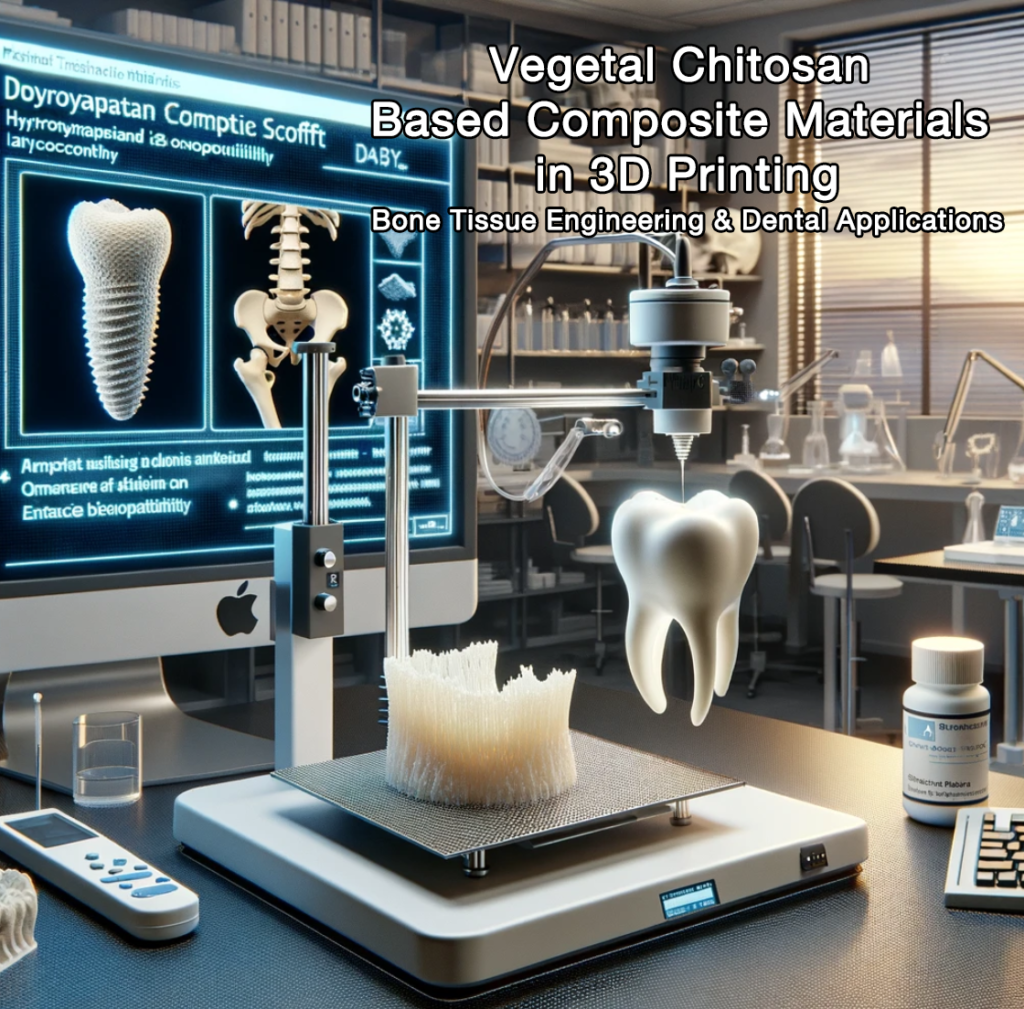
Each of these forms allows chitosan to be utilized effectively in 3D printing applications, capitalizing on its biocompatibility, biodegradability, and functional properties. The choice of form depends largely on the specific application and the type of 3D printing technology being used.
And each form of chitosan is tailored to maximize its benefits in specific 3D printing applications, leveraging its biocompatibility, biodegradability, and functional customization to meet the demanding needs of medical and biotechnological fields.
7. What is the flowchart of vegetal chitosan processing?
The production process of plant chitosan is mainly obtained by extracting raw materials (mushrooms, Aspergillus niger), deproteinizing with dilute acid or alkali, deacetylating, drying, etc.
Here is a simplified flowchart of the production process of vegetal chitosan for your reference.
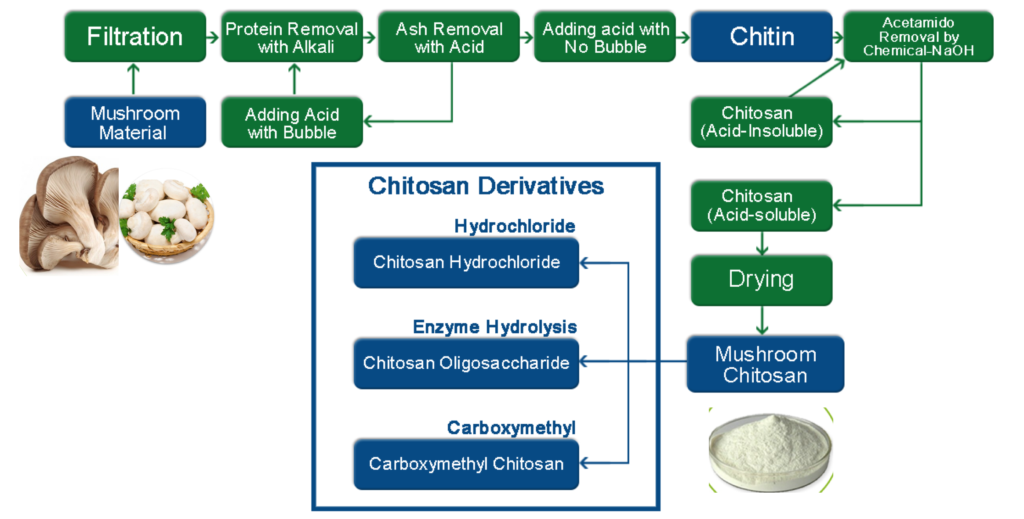
The flowchart of mushroom chitosan illustrates the process of producing chitosan and its derivatives from mushroom material. Here’s a summary of the key content:
- Starting Material: The process begins with mushroom material as the source.
- Filtration: The mushroom material undergoes a filtration process.
- Protein Removal: Proteins are then removed from the filtered material using an alkali solution.
- Ash Removal: Ash content is subsequently removed with acid.
- Chitin Extraction:
- Acid is added without bubbles to proceed to the next stage.
- Chitin is extracted, which is not soluble in acid.
- An acetylation step removes the acetyl groups from the chitin using sodium hydroxide (NaOH), converting it into chitosan, which is soluble in acid.
- Drying: The acid-soluble chitosan is then dried to produce the final mushroom chitosan product, showcased as a white powder.
- Chitosan Derivatives: Parallel to the drying process, there is a branch leading to the production of various chitosan derivatives:
- Chitosan Hydrochloride: Chitosan converted into its hydrochloride form.
- Enzyme Hydrolysis: Produces chitosan oligosaccharide through enzymatic hydrolysis.
- Carboxymethyl Chitosan: Derived through the carboxymethylation of chitosan.
The flowchart depicts a methodical approach to converting mushroom material into various forms of chitosan, focusing on the purification and chemical modification steps necessary to achieve different chitosan-based products for use in various applications.
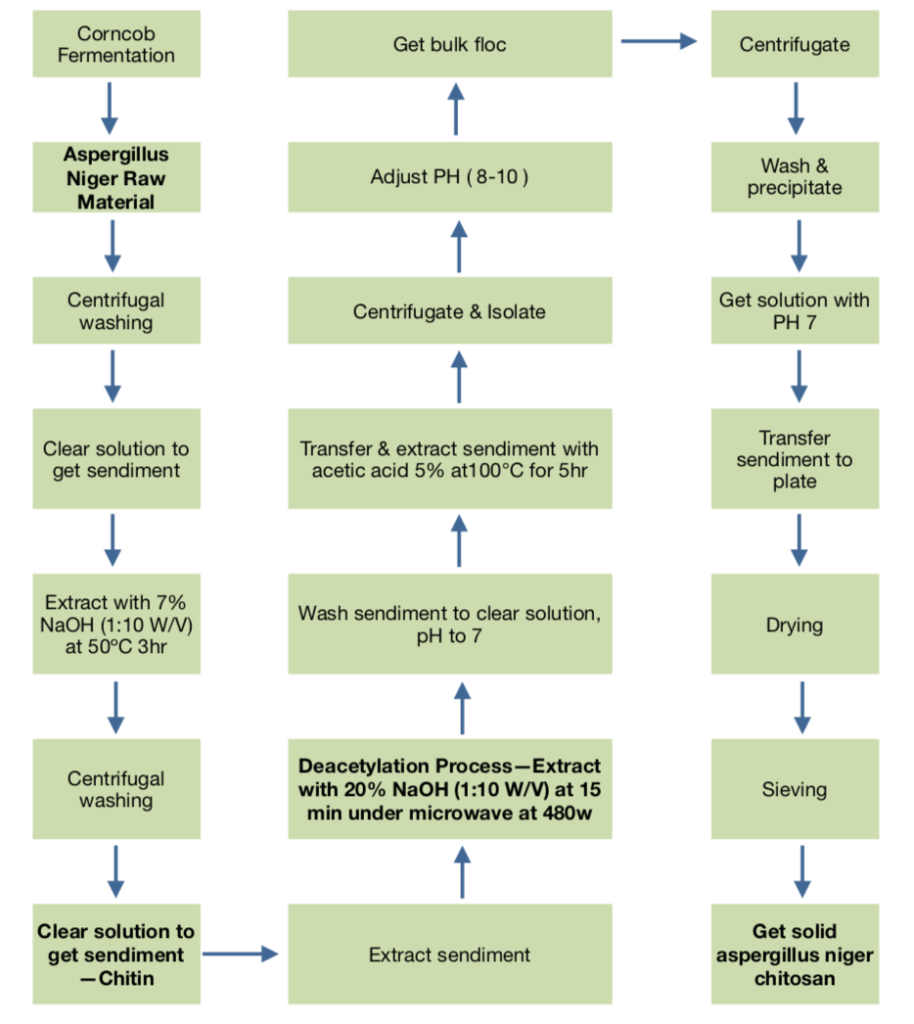
The flowchart of aspergillus niger chitosan outlines the process for extracting chitosan from Aspergillus niger, a type of fungus. Here’s a step-by-step summary of the key points:
- Starting Material: Aspergillus niger is cultured through a fermentation process using corn.
- Extraction:
- The fungal biomass undergoes a bulk flocculation.
- This is followed by centrifugation to separate the components.
- The pH of the resulting material is adjusted to alkaline conditions (pH 8-10).
- Another round of centrifugation and isolation occurs.
- The pH is adjusted back to neutral (pH 7), and the sediment is washed and precipitated.
- The sediment is then extracted with 5% acetic acid at 100°C for five hours to get the clear solution.
- It is washed again to achieve a clear solution with a neutral pH.
- Chitin Production:
- The clear solution is treated with 7% sodium hydroxide (NaOH) at a ratio of 1:10 (W/V) at 50°C for three hours.
- Centrifugal washing follows, resulting in the production of chitin as a clear solution to get the sediment.
- Deacetylation:
- The sediment undergoes the deacetylation process. It’s treated with 20% NaOH at a ratio of 1:10 (W/V) and heated in a microwave at 480W for 15 minutes.
- This step is crucial to convert chitin into chitosan by removing acetyl groups.
- Final Steps:
- The final sediment, which is now deacetylated chitin or chitosan, is extracted.
- It undergoes drying, followed by sieving to achieve the desired particle size.
- End Product: The final product is solid Aspergillus niger chitosan.
This process includes several steps involving pH adjustment, centrifugation, chemical treatments, and heating, which are critical to ensuring the purity and quality of the chitosan extracted from Aspergillus niger.
8. Summary of of vegetal chitosan used in 3D printing application
Vegetal chitosan, derived from sources such as mushrooms and Aspergillus niger, is increasingly recognized as a pivotal material in the realm of 3D printing, particularly within biomedical applications.
This naturally occurring biopolymer is lauded for its exceptional biocompatibility, biodegradability, and antimicrobial properties, making it an ideal candidate for creating complex tissue scaffolds, drug delivery systems, and customized prosthetics.
The ability of vegetal chitosan to form hydrogels and be processed into powders or filaments enhances its utility in various 3D printing technologies, allowing for precise customization to meet specific medical needs.
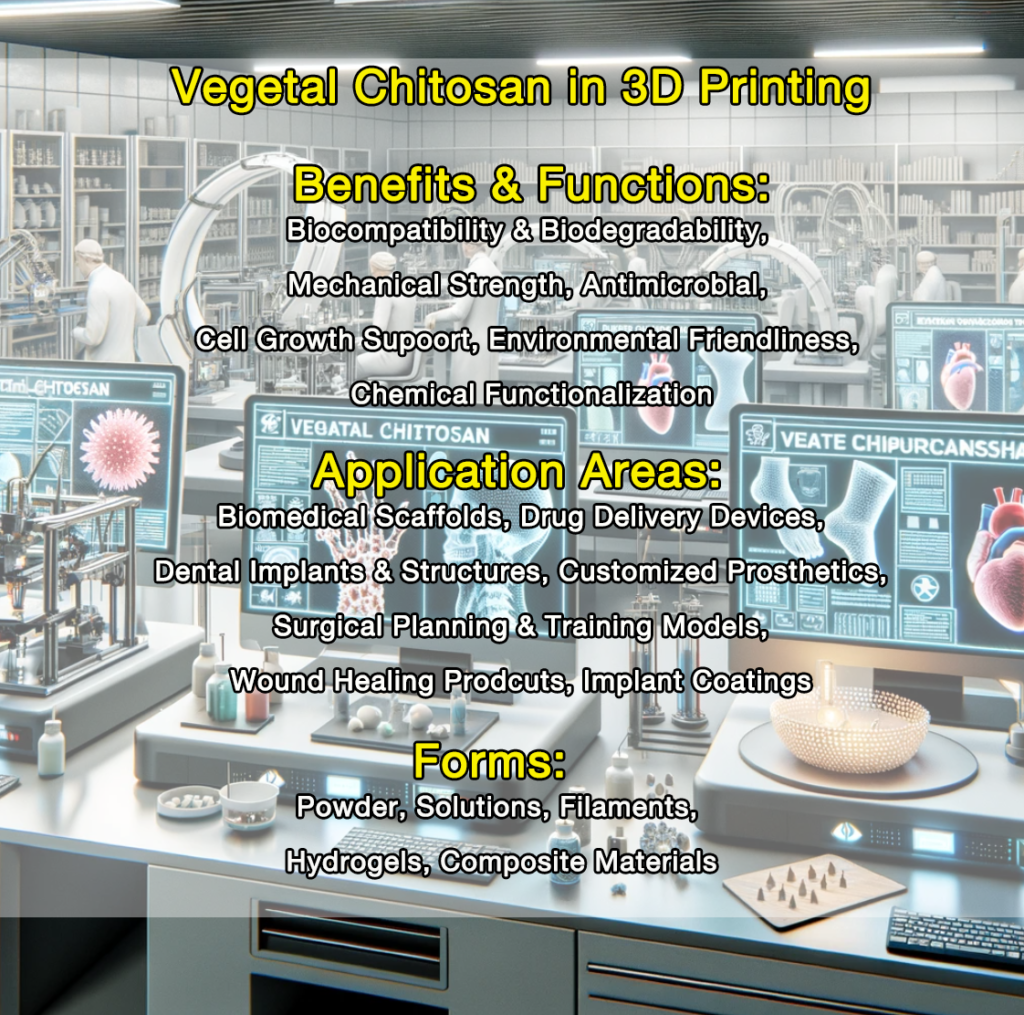
Its versatility is further highlighted in applications requiring direct contact with biological tissues, where its natural origin significantly reduces the risk of adverse reactions, promoting safer and more effective clinical outcomes.
In the dynamic field of 3D bioprinting, vegetal chitosan stands out for its adaptability and functionality.
By combining it with other biocompatible materials, researchers can engineer composite biomaterials that mimic the natural extracellular matrix, supporting cellular activities and encouraging tissue regeneration.
Whether it’s crafting detailed anatomical models for surgical preparation, designing scaffolds that support the growth of bone or cartilage, or formulating responsive drug delivery mechanisms, vegetal chitosan contributes significantly to advancements in medical technology.
The environmental sustainability of sourcing chitosan from fungi, as opposed to traditional marine sources, further enhances its appeal in modern biomanufacturing, aligning with global efforts towards greener production practices and reduction of carbon footprints in medical material production.